Project descriptions
The nuclear physics research group at the University of Manchester is one of the largest in the UK. The group consists of six academic staff with a variety of research interests.
Most of our current research is carried out by performing experiments at major international facilities, such as at Argonne National Laboratory (USA), CERN (Switzerland), Jyvaskyla (Finland), GANIL (France), GSI (Germany), and ILL reactor at Grenoble.
Magnetic Properties of Heavy Fission Fragments
Supervisor: Dr Gavin Smith
Using detailed measurements of perturbed angular correlations between gamma rays it is possible to extract both g-factors and M1/E2 mixing ratios. This project uses the GAMMASPHERE array to perform such measurements in fission fragments around Z=56. In parallel with this work the student will develop a novel piece of apparatus for the measurement of g-factors of very short-lived excited states.
Laser Spectroscopy of Exotic Nuclei
Supervisor: Dr Kieran Flanagan
Measurements of optical isotope shifts and hyperfine structures by laser techniques provide some of the most basic properties of radioactive nuclei. This type of measurement is particularly powerful since it does not rely on any assumptions of a particular nuclear model and therefore can be used to rigorously test theoretical predictions. Even more fundamentally, an optical resonance can confirm the simple fact of existence of a nuclide ground state or isomer. A key component of our research is the further improvement of the sensitivity of laser techniques in order to study these properties as far from stability as possible in order to approach r- and rp-process nuclei.
The student will participate in the development of a new technique of collinear resonance ionization spectroscopy at ISOLDE-CERN and at the PSI Manchester. The student will utilize techniques from quantum optics to further increase the resolution and sensitivity of the experiment. Development work will be carried out at the PSI before the techniques are used to study unstable nuclei at CERN. The student is expected to spend a significant part of their PhD at CERN on a Long Term Attachment (LTA). The student will receive training in state-of-the-art nuclear theory through the UK Nuclear Graduate School and international schools as part of the PhD.
Laser Preparation of Cooled Radioactive Atoms
Supervisor: Dr Paul Campbell
Our group works in a collaboration that performs laser spectroscopic studies of the nucleus. Experimental work is based at an international laboratory (JYFL) in Finland. Thesis studies undertaken in the laser collaboration at JYFL will concentrate on the effects of, and experimental opportunities afforded by, optical pumping in an on-line ion trap (that captures and holds short-lived radioactive ions). The studies will aim to provide experimental techniques for the manipulation of state and sub-state populations in ionic ensembles and will use the manipulated ionic populations to study new nuclear phenomena.
Optical pumping effects in radioactive ion traps have only recently been demonstrated to be significantly strong and a novel experimental technique awaits development and exploitation. In detail, the student will develop laser techniques capable of manipulating the ground and metastable state populations in ionic ensembles and use this technique to enable the on-line spectroscopic study of previously inaccessible nuclear species. The student will also develop techniques for the manipulation of magnetic substate populations in radioactive ionic ensembles and use these techniques for the study of polarised radioactive ion beams.
Exposing subtle details of the nuclear force through lifetime measurements
Supervisor: Dr David Cullen
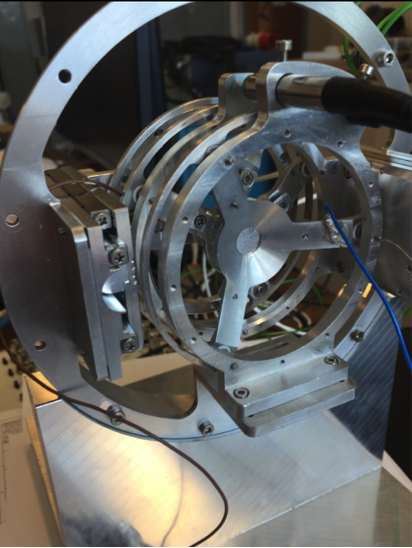
The lifetime of a nuclear state is a fundamental observable which gives a direct probe of the nuclear force. Classically, the lifetime, T, is given by the quantum mechanical Fermi-Golden rule,
where H is the nuclear Hamiltonian, ψi and ψf are the initial and final nuclear states involved in the process and ρ(E)dE is the density of final states. For nuclei, the transition between the initial and final states usually takes place with the emission of an electromagnetic gamma-ray which conserves angular momentum, parity and energy. Measurements of the nuclear state lifetimes in exotic nuclei allow subtle details of the nuclear force to be examined far from stability where weak but important parts of the nuclear force are often revealed. Lifetime measurements remain the best way to deduce the presence or absence of nuclear collectivity necessary to determine the exact location of new shell closures in exotic nuclei.
With STFC support we have developed and built a highly efficient Differential Plunger for Unbound Nuclear States (DPUNS) [1].
DPUNS has allowed progress to be made in the understanding of excited state lifetimes in exotic nuclei beyond the proton drip line. Several experimental campaigns have been performed in Finland with over 30 publications over a three-year period with 3-completed and 2-current PhD theses. The PhD students are expected to lead the research analysis and are first authors on all of the published papers. The next DPUNS campaign of 4 experiments will take place in Finland in May 2017.
Our recent research focus has been on:
- Defining the location of closed shells:
The lifetime of the nuclear states directly defines the nuclear collectivity. This gives information on how a quantum-mechanical ensemble of ~200-300 strongly interacting nucleons acts together to produce very large transition rates, orders of magnitude greater than the single-particle values. The determination of nuclear collectivity is extremely important around closed shells where collectivity must fall to zero and single-particle transition rates should be observed.
The N=Z=50 Closed Shell: We have studied this effect around the doubly magic N=Z=50 closed shell by measuring lifetimes in 109I and 112Te. These nuclei appear to show anomalies in the trend of decreasing collectivity as the doubly-magic closed shell is approached [2]. This may constitute evidence for an isospin dependence of the effective charges predicted by Bohr and Mottelson in 1975. We are currently testing these ideas with new lifetime data on 113Te and 112I.
The Z~114 Closed Shell: In order to radically understand the role of spin-orbit interaction in the stabilisation of super-heavy nuclei, and to determine the location of the highest N,Z shell closures, we have modified DPUNS to be used as a charge-plunger. This will allow a determination the lifetimes and collectivity of nuclear states in the super-heavy nuclei for the first time and thereby, define the location of the next proton shell gap after Z=82. Current state-of-the-art theoretical models currently predict very different values. Microscopic-macroscopic models predict Z=114 and mean-field approaches predict Z=120, 124 & 126. The main reason for these discrepancies is that the models rely on large extrapolations from regions where the interactions are experimentally verified. Our lifetime measurements of these nuclei will help validate the various theoretical models through a series of experiments on the nuclei above and around 252No (Z=102). DPUNS charge plunger will be commissioned in Finland late in 2017 and the main experiments performed in 2017-2019. A PhD student starting in September 2017 is ideally placed to lead the analysis of this project.
- A simultaneous understanding of proton- and gamma-ray emission in exotic nuclei beyond the proton drip line:
Over the last four years, we have used DPUNS to measure the lifetimes of unbound nuclear states in proton-emitting nuclei. With these measurements, we have developed a new theoretical framework which simultaneously treats proton emission and electromagnetic emission with a common set of wave functions [3]. The wave functions for the unbound states, Ψi and Ψf are derived from a Woods-Saxon model which considers the odd-proton to be orbiting a core which is effectively given by the wave function of the daughter nucleus, Ψd. For a given deformation (deduced from our lifetime measurements) the code therefore, simultaneously predicts both the parent and daughter wave functions, ψp and ψd, which are then fixed and used in Fermi-Golden rule expressions with Hamiltonians for both particle-emission with and electromagnetic transition rates .
We have used this theoretical approach to understand proton emission across a range of nuclei with very different deformations where the proton is coupled both adiabatically and non-adiabatically to the core. This approach has allowed a better description of proton emission process. We have used this approach for the mildly deformed oblate proton emitter 151Lu. Not only was this the best evidence to date for proton emission from an oblate nucleus, it also settled a long-standing 30-year theoretical debate on the shape of this nucleus [3,4]. This year, we finalised lifetime measurements for the deformed 113Cs. These results were used within the theoretical framework to simultaneously determine that both proton emission and gamma-emission from 113Cs are best described with a relatively large quadrupole deformation [5]. We are currently expanding this programme with STFC funding to be able to study more exotic nuclei with the development of a new triple-foil plunger for exotic nuclei (TPEN).
This device will allow lifetimes to be measured in more exotic nuclei with smaller production cross sections for the first time. TPEN will be commissioned in May 2017 in Finland. A PhD student will lead the data analysis of this commissioning experiment in 2017 and TPEN will later (2018-20) be used with radioactive beam developments at CERN, RIKEN (Japan) and FAIR (Germany).
References:
[1] M. J. Taylor, D. M. Cullen, et al. Nuclear Instr. and Methods in Phys. Res. A707 (2013) 143.
[2] M.G. Procter, D. M. Cullen, et al., Physics Letters B 704 (2011) 118.
[3] M. G. Procter, D. M. Cullen, M. J. Taylor et al., Physics Letters B 725 (2013) 79.
[4] M.J. Taylor, D.M. Cullen et al. Phys. Rev. C 86 (2012) 044310.
[5] D. Hodge, D.M. Cullen et al. PHYSICAL REVIEW C 94, 034321 (2016).
Contact: david.cullen@manchester.ac.uk
Improved Identification of illicit materials using an X-ray Backscattering technique
Supervisor: Dr David Cullen
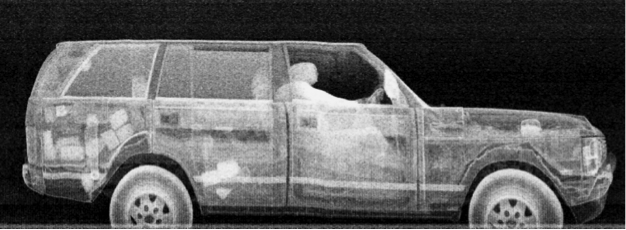
The aim of this research is to demonstrate the potential improvements that can be made in X-ray backscattering techniques to better identify illicitly smuggled material in cargo / baggage. The possibilities of this project will be achieved by combining a detailed understanding of the X-ray scattering processes and Monte-Carlo modelling with experimental results from poly-energetic X-ray sources and new high-efficiency, high-resolution CZT detectors.
Current state-of-the-art commercial X-ray backscatter cargo scanning systems make use of the increased scatter that results from low-Z (atomic number) organic materials to identify contraband items. The detectors used in current systems are only capable of measuring the intensity of scattered X-rays. However, areas of high-backscatter intensity, typically associated with contraband (drugs, tobacco, plastic explosives & currency), can be established as brighter regions in an X-ray image. Figure 1 shows a typical X-ray backscatter image of a car being driven through a scanner with contraband items hidden within the body panels.
This rather basic level of organic/inorganic separation is usually the only information available. To date, this has been considered sufficient to identify crates which don’t meet their manifest and require human inspection. This process creates delays and some crates are unnecessarily opened.
Working together, Manchester University and Rapiscan systems (Cheshire) recently demonstrated that more information relating to the material composition of the inspected object was available within backscattered X-ray data. By measuring both the intensity and energy of the backscattered X-rays simultaneously it was possible to increase the level of material separation beyond that of simple inorganic/organic. The key aspects of this project were the unique combination of new high-resolution, high-efficiency, CZT detectors coupled with validation and comparison of the experimental measurements with theoretical simulations using the Geant IV (Monte-Carlo) code. In this project, the original setup will be scaled up to evaluate whether this approach can be a realistic option for an improved large-scale industrial cargo security screening device. A new CZT multi-detector setup will be used with higher-intensity poly-energetic X-ray sources, first with a pulsed 50-keV table-top X-ray source at The University of Manchester and later with a larger 200-keV X-ray source at Rapiscan systems in Cheshire.
A fully-funded STFC-CASE 3.5 year PhD studentship is currently available for this project which includes an additional stipend and 1 year based at Rapiscan systems in Cheshire.
Molecular Radiotherapy Dosimetry
Supervisor: Dr David Cullen
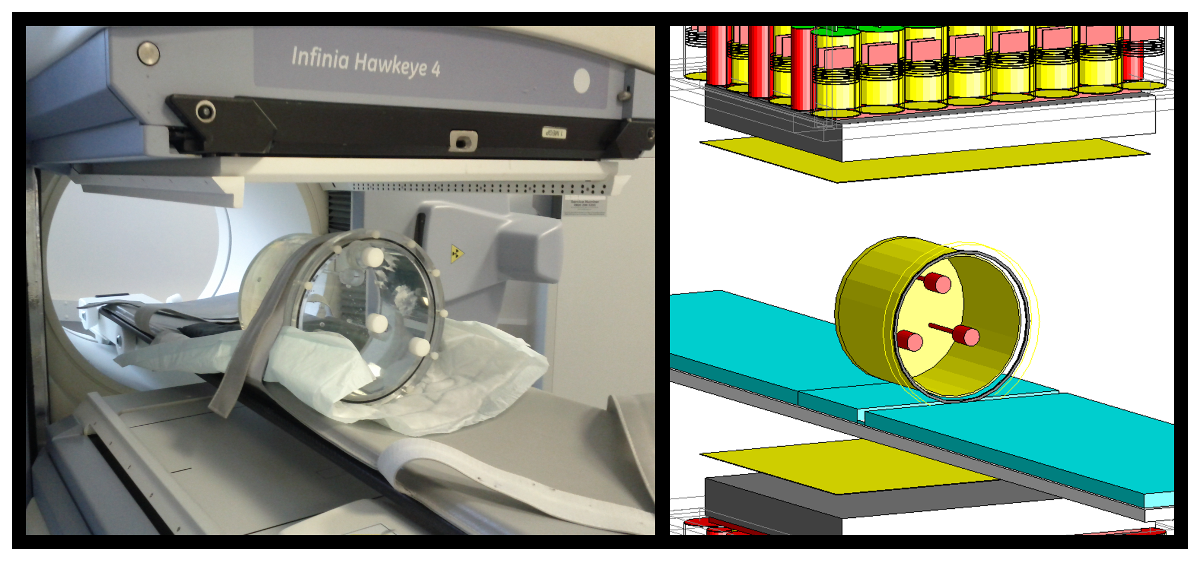
Molecular Radiotherapy (MRT) is a cancer therapy technique in which radioactive pharmaceuticals are administered inside the body to deliver a lethal radiation dose to malignant cells whilst sparing surrounding healthy tissue. There is now an extensive clinical portfolio of MRT procedures which has been significantly enhanced with the introduction of new radiopharmaceuticals. MRT treatment is generally minimally invasive, incurs few side effects and can achieve impressive results.
When delivering MRT, the radiation doses to the tumour and normal tissue should be accurately determined for each patient using nuclear-medicine imaging - an essential component in the diagnosis, staging and management of many oncological conditions. Due to the inherent complexity of measuring radiation doses from in vivo decays current MRT dose calculations are still non-optimised generic averages based on theoretical organ dimensions without regard for individual patient size and weight. Ultimately because of the uncertainty in determining doses for radiation sensitive organs, a significant proportion of patients do not receive critical tumour doses and therefore, the greatest therapeutic benefit.
Our collaboration between the Manchester nuclear-physics group and the nuclear-medicine group at The Christie NHS Foundation Trust has established a fruitful interdisciplinary research program which seeks to address the complex and interconnected problem of providing accurate patient specific dosimetry for MRT.
Our research focuses on two key aspects of MRT dosimetry:
- The application of Monte-Carlo simulation techniques to improve activity quantification and dosimetry calculations for a variety of MRT treatments.
- The validation of dosimetry calculations using realistic patient analogues.
By using a detailed Monte-Carlo simulation of a GE Infinia Hawkeye 4 SPECT/CT camera (as used at The Christie) we can fully track gamma rays and beta particles interactions from the initial decay within a patient body until detection in the SPECT camera. Figure 1 shows our simulation which has been used to model SPECT scan acquisitions of simple water filled phantoms (approximating the human body), more complex anthropomorphic human shaped phantoms and CT images of patient therapies. By characterising the scattering of gamma rays in the SPECT camera system we have been able to significantly improve the image quality and accuracy of activity quantification.
Our research is focused on the development of 3D printing techniques to produce organ models to facilitate the validation of MRT dosimetry for realistic patient morphologies. These models allow many of the underlying systemic sources of error in MRT dosimetry calculations to addressed and provide a major step towards providing individualised patient-specific MRT treatment plans. The knowledge exchange and output [1-2] from this project has had direct impact at The Christie. We have been awarded several STFC-IPS grants over the past 6 years and the collaboration has been extended with a commercial partner HERMES Medical Solutions Ltd. This leading supplier of cross platform nuclear medicine software provides a pathway to translate our research into a commercial product. The group currently has three PhD students (two funded by an STFC CASE awards) and a postdoctoral researcher.
Publications:
[1] A P Robinson, D.M. Cullen et al. Phys. Med. Biol. 61 (2016).
[2] A P Robinson, D.M. Cullen et al. EJNMMI Phys 3 (1), 12. (2016).
Exploring the Changing Shell Structure of Nuclei
Supervisor: Professor Sean J Freeman
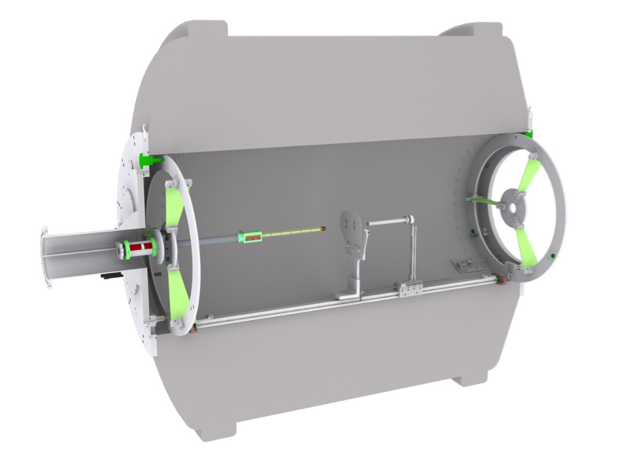
The introduction of the spin-orbit interaction by Maier and Jensen led to an understanding of the observed shell gaps and magic numbers in near-stable nuclei. The appearance of these ideas in undergraduate textbooks gives the impression of solidity and permanence to the well-known sequence of magic numbers. Recent observations, however, have challenged this basic assumption by suggesting that the sequence of single-particle states observed near stability is actually quite fragile; studies of nuclei far from the line of β stability have begun to indicate that the familiar shell gaps do not persist in exotic systems. Instead, shifts in the sequence of single-particle levels conspire to give gaps which change with changing nucleon number, fundamentally reshaping the basis of nuclear structure and producing new and unexpected phenomena. The reasons for these alterations to one of the basic tenets of nuclear physics are currently being debated and are of paramount interest in the development of the understanding of atomic nuclei.
Single-nucleon transfer reactions offer a suitable probe of the single-particle characteristics via the spectroscopic factor (SF), measuring the overlap of the wave function of a state with simple single-particle configurations. Being subject to sum rules, SFs allow access to the occupancies of underlying single-particle orbits.
Phd projects in this area will involve using transfer reactions to measure the single-particle energies of particular single-particle orbitals along chains of isotopes and isotones in order to track the evolution of single-particle structure. This may involve making measurements on stable targets as well as using radioactive beams in order to track single-particle structure away from stability. This work utilises novel devices such as the HELIOS spectrometer at Argonne National Laboratory, Chicago, USA. HELIOS has been designed for the study of transfer reactions using radioactive beams.
Nuclear observables relevant to neutrinoless double-beta decay
Supervisor: Professor Sean Freeman
In order to have confidence in any neutrino mass determined via the observation of neutrinoless double-beta decay it is important to validate the calculated nuclear matrix elements. Observation of 0νββ would allow the absolute neutrino masses to be determined if the associated matrix elements can be determined. No other physical process samples these directly and so complex calculations must be used to determine them. Different methods (QRPA, shell models, IBM etc.) have yet to reach satisfactory consistency. It is clear that the initial/final nuclear wave functions are important and transfer reactions are able to provide useful tests by benchmarking the relative occupation of valence orbitals. Some calculations use the BCS approximation, the validity of which can be assessed by pair transfer.
Phd projects in this area would involve using single-nucleon transfer reactions to measure the neutron and proton occupancies of neutrinoless double-beta decay candidates. These results can then be compared to theoretical predictions in order to validate the nuclear matrix element calculations relevant to neutrinoless double-beta decay. Additionally the measurement of pair-transfer can be used to validate the BCS approximation in these nuclei. These measurements are made at the Maier-Leibnitz Laboratorium, Munich, Germany using a tandem accelerator and the Q3D spectrometer as well as at RCNP, Osaka, Japan using coupled cyclotron accelerators and the Grand Raiden spectrometer.
Probing the Origin of Fission-Fragment Angular Momentum
Supervisor: Dr Gavin Smith
It is well known that the angular momentum vectors of fission fragments are aligned roughly perpendicular to the fission axis. This alignment means that gamma rays emitted from a fission fragment are correlated with the fragment direction. In addition, in spontaneous fission of an even-even nucleus, the initial angular momentum is zero which means that the fragment spins are correlated leading to anisotropy in the emission of inter- fragment gamma rays. Our measurements suggest that this correlation is not as strong as one might expect. Is this because of the role of relative angular momentum or is it because the spin vectors are tilted at scission? This project uses measurements of fragment-gamma and inter-fragment gamma-gamma correlations using data collected at GAMMASPHERE to try to answer these questions.
Pulse-shape Analysis with a Bragg-Curve Spectrometer
Supervisor: Dr Gavin Smith
Bragg-curve spectrometers are heavy-ion gas detectors that have an electric field applied along the trajectory of the ion. The gas is ionized along the ion track and drifts towards the anode. The anode signal contains information on the energy and atomic number of the ion. For fission fragments, the velocity is such that the previous crude method of fast/slow integration of the anode pulse is not adequate. We have now available fast electronics which allows the digitization of the signal. The current research project involves taking data with this system at Manchester, developing the algorithms to determine Z and E in real time and then using the system to do gamma-spectroscopy on highly exotic fragments of neutron-induced fission at the ILL High- flux Reactor facility in Grenoble, France.